Baumann Lab
Research Publications Group Members BiographyTelomere biology & chromosomal inheritance
I. Telomere maintenance
Elucidating the mechanisms by which chromosome ends are protected and maintained has far-reaching implications for the treatment of cancer and degenerative diseases. Telomere shortening is part of normal cell proliferation. In stem cells; some transiently dividing cells; and many cancers telomeric DNA is replenished by the enzyme telomerase, a ribonucleoprotein complex that uses part of a long noncoding RNA as a template for telomere synthesis. Our group isolated the telomerase RNA subunit (TER1) from fission yeast, which provided a key tool to study the enzyme telomerase in a genetically tractable organism. This led to the discovery that the RNA splicing machinery has a second, previously overlooked, function in RNA 3’ end processing. Instead of removing an intronic sequence in a two-step process, the first transesterification reaction alone generates the mature 3’ end of TER1. This work raised several questions that form the basis for ongoing projects: (i) Is TER1 the only target for spliceosomal cleavage or are any other non-coding RNAs processed by spliceosomal cleavage? (ii) What is the mechanism and evolutionary origin of spliceosomal cleavage? (iii) Is spliceosomal cleavage regulating gene expression via a programmed switch from splicing to cleavage?
Our research further revealed that Sm and LSm proteins sequentially associate with telomerase RNA and play distinct roles in telomerase biogenesis. There is considerable medical interest in these proteins as mutations in the survival of motor neuron (SMN) gene cause spinal muscular atrophy, a leading cause of infant mortality. It is known that SMN loads Sm proteins onto target RNAs, but why mutations cause progressive muscle degeneration is still unclear.
Ongoing studies are defining the roles of RNA chaperones in telomerase biogenesis and uncovering links between RNA processing and quality control in the regulation of telomerase. While our studies on telomerase and telomeres in fission yeast are providing fundamental insights into chromosome end maintenance, we are also interested in understanding the biogenesis and regulation of the human enzyme. Recent work in our lab has uncovered roles for the cap-binding complex, the nuclear exosome-targeting complex, the Trf4/Air2/Mtr4 polyadenylation complex, the exosome, and poly(A) ribonuclease in the biogenesis of human telomerase. We further showed that human telomerase RNA (hTR) processing is in kinetic competition with degradation, an observation that hints at potential treatment options for telomerase insufficiency disorders. These results will guide future studies aimed at identifying additional factors involved in telomerase RNP biogenesis. Overall, our research goals in this area are guided by the conviction that a better understanding of telomerase biogenesis, and the dynamic interactions that occur at telomeres, will enable us to identify compounds that modulate telomere length. Such reagents will have therapeutic use either to limit the lifespan of tumour cells or to boost the proliferative potential of desired cell populations.
II. Hybridization, ploidy & parthenogenesis
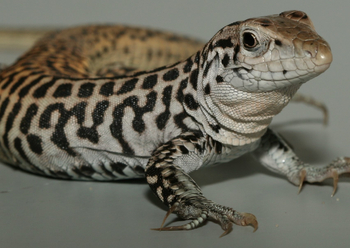
Our interest in chromosome dynamics and inheritance led us to a group of lizards that reproduce without males, and display a remarkable tolerance for changes in ploidy. At the time, little was known about the molecular mechanisms that permit females of some species to clone themselves by parthenogenesis. We addressed this with a series of experiments that revealed how female whiptail lizards ovulate unfertilized eggs carrying the full chromosome complement without loss of heterozygosity. We are now using a combination of ovarian tissue culture, confocal microscopy and electron microscopy to gain insights into the cellular and molecular mechanisms through which barriers to unisexuality are overcome.
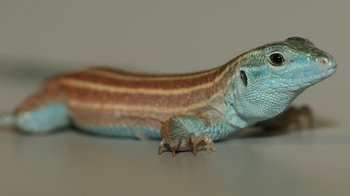
Interspecific hybridization endows clonal lineages with high levels of heterozygosity and plays a key role in the transition from sexual to parthenogenetic reproduction in vertebrates. Ploidy elevation further increases heterozygosity as the additional genome expands the genetic repertoire that may permit adaptation to a changing environment or the colonization of new ecological niches. Indeed, two-thirds of parthenogenetic species of whiptail lizards are triploids and many of the diploid intermediates are thought to be extinct. Taking advantage of our laboratory colony, we have produced over 100 hybrids between sexual males and parthenogenetic females. These experiments resulted in the first tetraploid lizards that have founded new genetically isolated lineages. The availability of sterile hybrids and clonally reproducing lineages has opened a new angle for investigating the molecular basis of parthenogenetic reproduction and the causes for hybrid sterility. We will compare sterile and fertile animals using cytological and molecular approaches to decipher the elements that block parthenogenesis in some hybrids but not others. We have already sequenced and assembled the genomes of three species of whiptail lizards and are in the process of developing a comprehensive genomic toolkit to shed light on the following topics:
(i) In evolutionary biology, much has been written about the process through which deleterious mutations accumulate in the genomes of asexual species to create a genetic load (Muller’s ratchet) and the need for continuing adaptation (the red queen concept). These hypotheses form the framework for explaining why a lack of genetic diversity and limited ability to evolve would condemn obligate unisexuals to extinction. However, an important issue that has received little attention in the debate is the actual assessment of genetic (and epigenetic) diversity that exists in unisexual populations despite “clonal” reproduction. We have conducted a small-scale study of microsatellites variability and found that some loci varied as much within a parthenogenetic species as they did in sexual species. We will derive a far more comprehensive understanding of the degree of diversity, mutation accumulation and epigenetic variation from the analysis of next-generation sequencing data.
(ii) The hybrid origin of parthenogenetic species is often cited as a key element in their long-term success. But combining genomes from two or more species in one organism can also result in incompatibilities. The side-by-side comparison of first-generation hybrids with parthenogenetic individuals, that originated from the same parental species in nature many generations ago, provides a unique opportunity to examine adaptation to the presence of divergent genomes in the same cell and organism. We will compare allele-specific gene expression in laboratory-synthesized hybrids with individuals of parthenogenetic species known to harbour the same two genomes for thousands of generations. These studies will provide insights into how allele-specific gene expression is established, fixed and maintained over time.
Our lizard colonies together with genomic resources generated by our group provide a unique opportunity to elucidate the molecular basis of unisexual reproduction and to understand the barriers that limit parthenogenesis in most vertebrates. Such research carries the promise of furthering our knowledge of evolution and gene regulation in the context of hybridization, ploidy variation and parthenogenesis.
For more information, please visit the Baumann Lab website.